News
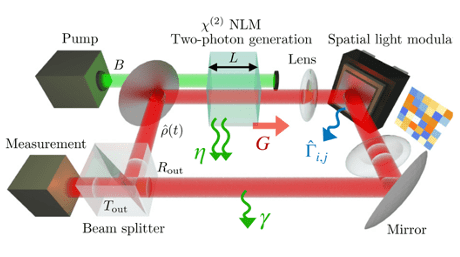
Emergent Equilibrium in All-Optical Single Quantum-Trajectory Ising Machines
A collaboration between the Theory group at MPQ, CREF Rome, and Sapienza University of Rome has shown that multimode all-optical systems driven by two-photon processes can reach thermal equilibrium at the level of single quantum trajectories. The study is published in...
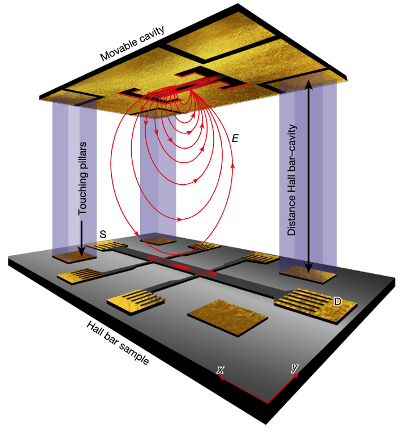
Cavity-enhanced fractional quantum Hall phases and cavity-modified spin splittings
A collaboration between the Equipe Théorie at MPQ and scientists from ETH Zurich and the Flatiron Institute has demonstrated, both theoretically and experimentally, that giant vacuum fields confined in a split-ring resonator cavity can profoundly alter...
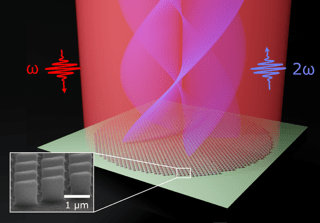
Unravelling the nonlinear generation of designer vortices with dielectric metasurfaces
Researchers from the DON team have demonstrated and analyzed the generation of high-purity second-harmonic vortices with dielectric metasurfaces. These results are published in Light: Science and Applications. Vortex beams are currently drawing a great deal of...
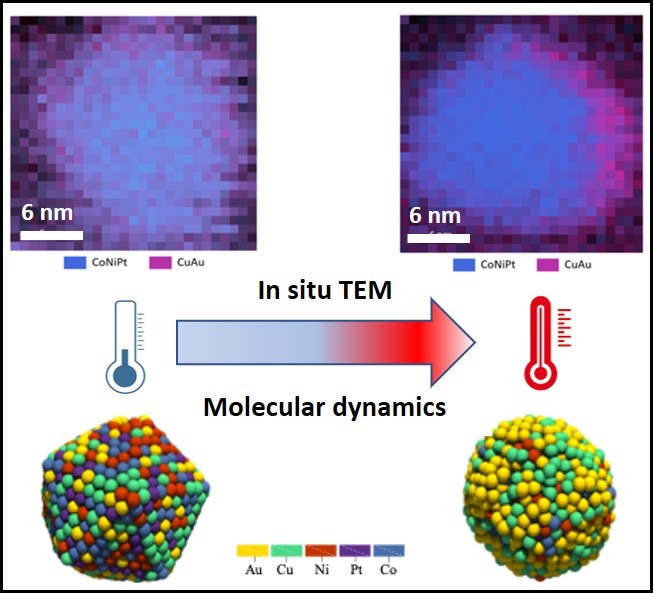
Thermal stability of high-entropy nanoalloys: reality or chimera?
By studying at the atomic scale the thermal behavior of nanoparticles composed of gold, cobalt, copper, nickel and platinum, scientists have revealed that the stability of these so-called high-entropy nanoalloys is much lower than expected, as gold and copper...
Nonlinear Optical Devices (DON)
Quantum Information and Technologies (QITE)
Theory group (THEORIE)
Light and Mechanics (LIME)
Advanced Electron Microscopy and Nano-Structures (MEANS)
Scanning Tunnelling Microscopy (STM)